Biomedical applications of electroactive polymer actuators
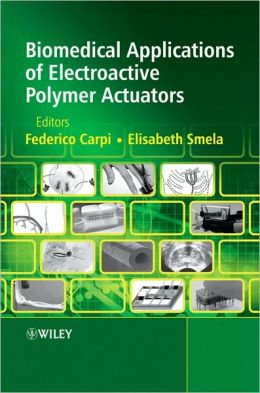
Preface
The great majority of traditional actuation technologies are based on thermochemical
(combustion) motors, electromagnetic drives and hydraulic/pneumatic machines.
However, these are inadequate to satisfy the diversity of new challenges presented in fields
such as mechatronics, robotics and biomedical engineering. The biomedical field is
particularly sensitive to the need for new types of actuators, since it includes applications
from the nanoscale through to the macroscale, with requirements that differ enormously in
terms of both structure and function. As an example, actuation devices span those designed
to interact with single cells, and potentially organelles and even molecular structures, up to
those used to replace limbs or to perform tele-operated surgery. Clearly, these different
areas of application require different forces, displacements and speeds, as well as different
durability, robustness and types of biocompatibility. Devices for cell manipulation might
be single-use and disposable, whereas artificial hearts must sustain billions of cycles.
New actuation materials and technologies should ideally have high work output, actuation
strain, mechanical compliance, damage tolerance and efficiency. Depending on the
application, they may also be required to be lightweight, have compact and simple
structures that can readily be fabricated, and be reasonably low cost. In most scenarios,
these new technologies will serve a complementary role working together with conventional
actuators.
In the last few decades this need for new actuators has drawn considerable effort
towards the development of materials that can directly transduce an input energy into
mechanical work. Much of this attention has been focused, and is increasingly being
focused today, on electroactive polymers (EAPs). This is a large family of materials
that includes many different chemical structures, actuation mechanisms and electromechanical
performances.
Although most EAP materials have been known for decades, before now they found
limited translation from proof of concept demonstrations in the laboratory to actual use,
despite their potential. This has changed with recent developments in materials science,
processing, configuration design and driving strategies which are permitting serious efforts
towards concrete exploitation, as this book describes. In fact, EAPs are opening the way to
numerous new applications precluded by conventional actuation technologies.
This book intends to provide a comprehensive and updated insight into both the fundamentals
of each class of EAP, and examples of the most significant applications of EAP
actuators in the biomedical field, either already demonstrated or currently under development.
For this purpose, the book comprises five sections devoted to the most technologically
mature EAPs, namely polymer gels, ionic polymer–metal composites, conjugated
polymers, piezoelectric/electrostrictive polymers and dielectric elastomers. Each section is
introduced by a chapter that is focused on the fundamentals and which aims to provide a
description of the main features of the technology and the current state of the art. These
introductory chapters are followed by chapters describing specific applications.
The contributors to this book are inventors and international leaders in the field.
The broad and far-reaching range of applications covered by this book is intended not
only to make it the first text on biomedical uses of the emerging EAP based actuation
technologies, but also to serve as a source of inspiration for possible new applications
aimed at improving health and well-being.
Federico Carpi, University of Pisa
Elisabeth Smela, University of Maryland
October 2008
Download
*
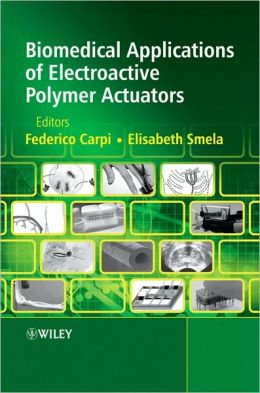
Preface
The great majority of traditional actuation technologies are based on thermochemical
(combustion) motors, electromagnetic drives and hydraulic/pneumatic machines.
However, these are inadequate to satisfy the diversity of new challenges presented in fields
such as mechatronics, robotics and biomedical engineering. The biomedical field is
particularly sensitive to the need for new types of actuators, since it includes applications
from the nanoscale through to the macroscale, with requirements that differ enormously in
terms of both structure and function. As an example, actuation devices span those designed
to interact with single cells, and potentially organelles and even molecular structures, up to
those used to replace limbs or to perform tele-operated surgery. Clearly, these different
areas of application require different forces, displacements and speeds, as well as different
durability, robustness and types of biocompatibility. Devices for cell manipulation might
be single-use and disposable, whereas artificial hearts must sustain billions of cycles.
New actuation materials and technologies should ideally have high work output, actuation
strain, mechanical compliance, damage tolerance and efficiency. Depending on the
application, they may also be required to be lightweight, have compact and simple
structures that can readily be fabricated, and be reasonably low cost. In most scenarios,
these new technologies will serve a complementary role working together with conventional
actuators.
In the last few decades this need for new actuators has drawn considerable effort
towards the development of materials that can directly transduce an input energy into
mechanical work. Much of this attention has been focused, and is increasingly being
focused today, on electroactive polymers (EAPs). This is a large family of materials
that includes many different chemical structures, actuation mechanisms and electromechanical
performances.
Although most EAP materials have been known for decades, before now they found
limited translation from proof of concept demonstrations in the laboratory to actual use,
despite their potential. This has changed with recent developments in materials science,
processing, configuration design and driving strategies which are permitting serious efforts
towards concrete exploitation, as this book describes. In fact, EAPs are opening the way to
numerous new applications precluded by conventional actuation technologies.
This book intends to provide a comprehensive and updated insight into both the fundamentals
of each class of EAP, and examples of the most significant applications of EAP
actuators in the biomedical field, either already demonstrated or currently under development.
For this purpose, the book comprises five sections devoted to the most technologically
mature EAPs, namely polymer gels, ionic polymer–metal composites, conjugated
polymers, piezoelectric/electrostrictive polymers and dielectric elastomers. Each section is
introduced by a chapter that is focused on the fundamentals and which aims to provide a
description of the main features of the technology and the current state of the art. These
introductory chapters are followed by chapters describing specific applications.
The contributors to this book are inventors and international leaders in the field.
The broad and far-reaching range of applications covered by this book is intended not
only to make it the first text on biomedical uses of the emerging EAP based actuation
technologies, but also to serve as a source of inspiration for possible new applications
aimed at improving health and well-being.
Federico Carpi, University of Pisa
Elisabeth Smela, University of Maryland
October 2008
Download
*