Neutron Scattering in Biology - Fitter Gutberlet and Katsaras
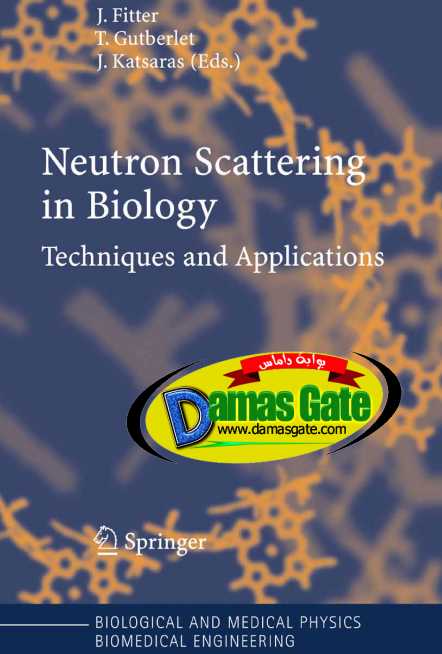
Preface
“Certainly no subject or field is making more progress on so many fronts at
the present moment, than biology, and if we were to name the most powerful
assumption of all, which leads one on and on in an attempt to understand
life, it is that all things are made of atoms, and that everything that living
things do can be understood in terms of the jigglings and wigglings of atoms.”
Richard P. Feynmann, from “Six easy pieces” (1963)
In 1932, James Chadwick discovered the neutron, but initially the only sources
of neutrons were from the radioactive decay of unstable nuclei. It was not until
1942 when Enrico Fermi constructed the first nuclear reactor in the squash
courts beneath the University of Chicago’s Stagg Field, that a controlled and
sustained nuclear chain reaction was achieved. After World War II, nuclear
reactors became available for civilian research, and in 1945 Ernest Wollan set
up a double-crystal diffractometer at ORNL’s Graphite Reactor. This marks
the beginning of neutron scattering.
Neutrons produced by present reactor- and accelerator-based sources,
typically have wavelengths in the order of
˚
Angstroms, and hence are well-
suited for probing the structures and motions of molecules. For biological
materials rich in hydrogen, the large difference in scattering cross-sections
between hydrogen and deuterium provides the possibility of contrast variation,
a powerful method achieved by selective deuteration for emphasizing,
or not, the scattering from a particular portion of a molecule or molecular
assembly. Using a variety of scattering methods, the structures and dynamics
of biological systems can be determined.
of biological systems can be determined.
The present compilation aims to provide the reader with some of the
important applications of neutron scattering in structural biology, biophysics,
and systems relevant to biology.
The location of hydrogen atoms in biomolecules such as, proteins,
is – despite the high brilliance and power of third generation synchrotron
sources – not readily available by X-ray crystallography or related physical
techniques. In the case of hydrogens attached to electronegative atoms (e.g.,
O and N), even high resolution X-ray structures (resolution
unequivocally locate these H atoms. On the other hand, these atoms can
effectively be located using high resolution crystallographic neutron diffraction
methods. Radiation damage leading to changes in metal oxidation state
and subsequent loss of hydrogens can also pose a problem with X-rays, but not
so with neutrons. When good quality, large (>1mm
) single crystals cannot
be obtained, low resolution neutron diffraction offers an alternative technique
in determining the hydrated structure of macromolecules and their various
hydrogen-bonding patterns. 3˚ A) cannot
Download
*
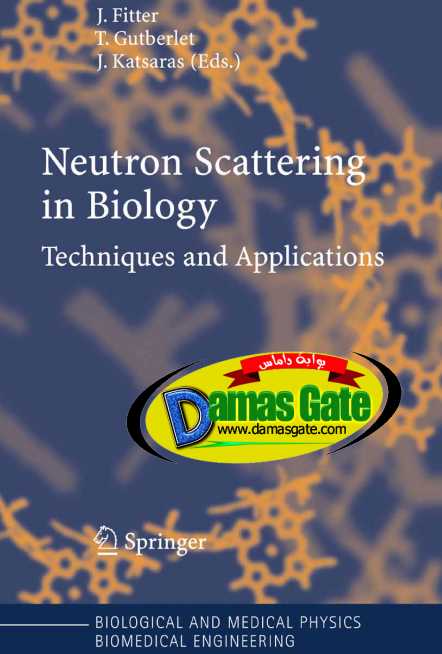
Preface
“Certainly no subject or field is making more progress on so many fronts at
the present moment, than biology, and if we were to name the most powerful
assumption of all, which leads one on and on in an attempt to understand
life, it is that all things are made of atoms, and that everything that living
things do can be understood in terms of the jigglings and wigglings of atoms.”
Richard P. Feynmann, from “Six easy pieces” (1963)
In 1932, James Chadwick discovered the neutron, but initially the only sources
of neutrons were from the radioactive decay of unstable nuclei. It was not until
1942 when Enrico Fermi constructed the first nuclear reactor in the squash
courts beneath the University of Chicago’s Stagg Field, that a controlled and
sustained nuclear chain reaction was achieved. After World War II, nuclear
reactors became available for civilian research, and in 1945 Ernest Wollan set
up a double-crystal diffractometer at ORNL’s Graphite Reactor. This marks
the beginning of neutron scattering.
Neutrons produced by present reactor- and accelerator-based sources,
typically have wavelengths in the order of
˚
Angstroms, and hence are well-
suited for probing the structures and motions of molecules. For biological
materials rich in hydrogen, the large difference in scattering cross-sections
between hydrogen and deuterium provides the possibility of contrast variation,
a powerful method achieved by selective deuteration for emphasizing,
or not, the scattering from a particular portion of a molecule or molecular
assembly. Using a variety of scattering methods, the structures and dynamics
of biological systems can be determined.
of biological systems can be determined.
The present compilation aims to provide the reader with some of the
important applications of neutron scattering in structural biology, biophysics,
and systems relevant to biology.
The location of hydrogen atoms in biomolecules such as, proteins,
is – despite the high brilliance and power of third generation synchrotron
sources – not readily available by X-ray crystallography or related physical
techniques. In the case of hydrogens attached to electronegative atoms (e.g.,
O and N), even high resolution X-ray structures (resolution
unequivocally locate these H atoms. On the other hand, these atoms can
effectively be located using high resolution crystallographic neutron diffraction
methods. Radiation damage leading to changes in metal oxidation state
and subsequent loss of hydrogens can also pose a problem with X-rays, but not
so with neutrons. When good quality, large (>1mm
) single crystals cannot
be obtained, low resolution neutron diffraction offers an alternative technique
in determining the hydrated structure of macromolecules and their various
hydrogen-bonding patterns. 3˚ A) cannot
Download
*